Issue |
A&A
Volume 495, Number 2, February IV 2009
|
|
---|---|---|
Page(s) | L13 - L16 | |
Section | Letters | |
DOI | https://doi.org/10.1051/0004-6361/200811535 | |
Published online | 09 February 2009 |
LETTER TO THE EDITOR
Discovery of an old photoevaporating disk in
Orionis
E. Rigliaco1,2 - A. Natta1 - S. Randich1 - G. Sacco3
1 -
Osservatorio Astrofisico di Arcetri, INAF, Largo E. Fermi 5,
50125 Firenze, Italy
2 -
Università di Firenze, Dipartimento di Astronomia, Largo E. Fermi 2,
50125 Firenze, Italy
3 -
Osservatorio Astronomico di Palermo, INAF, Piazza del Parlamento 1, 90134 Palermo, Italy
Received 17 December 2008 / Accepted 2 February 2009
Abstract
The photoevaporation of circumstellar disks is a powerful process in the
disk dissipation
at the origin of the Orion proplyds.
This Letter reports the first detection of a photoevaporating disk
in the final but long-lasting phase of its evolution.
The disk is associated to a low-mass T Tauri member
of the Ori Cluster. It is
characterized by a very low (if any) accretion rate
and by a tenuous (
/yr) photoevaporation
wind, which is unambiguously detected in the
optical spectrum of the object. The wind
emits strong forbidden lines of [SII] and [NII]
because the low-mass star is close to a
powerful source of ionizing photons,
the O9.5 star
Ori.
Key words: stars: formation - accretion, accretion disks
1 Introduction
The evolution of circumstellar disks surrounding low-mass T Tauri stars (TTS in the following) is controlled by the interplay of different physical processes, which include viscous accretion onto the central star, photoevaporation by the stellar radiation field, and planet formation. If the low-mass star is sufficiently close to a more massive and hotter object, photoevaporation by this external source of high-energy radiation is also important because it can dominate the disk evolution.
The process of photoevaporation has been extensively discussed
(see, e.g. Hollenbach et al. PPIV; Dullemond et al. PPV). High-energy photons heat gas disks to temperatures
such that the thermal pressure exceeds the gravity from the central star.
The disk evaporates from outside-in, with a mass-loss
rate that decreases with time as the disk shrinks.
Most of the well-studied Orion proplyds
are caught in the first, short evolutionary phase, when
the mass-loss rate is very high (
/yr, Henney
et al. 1999).
We report in this Letter on the first detection of a proplyd in
a much
later evolutionary phase, when the mass-loss rate is 10-8
/yr.
The object (a low-mass TTS) is located in the star-forming region
Ori (age
Myr), which contains the massive quintuplet system
Ori.
The brightest star of this
system (spectral type O9.5) forms a large, low-density HII region (Habart
et al. 2005) and the bright PDR known as the Horsehead (Abergel et al. 2003).
The low background from the HII region and the relatively short distance
(
400 pc;
Mayne & Naylor 2008) allow us to detect the slow photoevaporated TTS wind and to measure its
optical line emission spectrum.
2 The T Tauri star SO587
2.1 Stellar properties
SO587 (also identified as R053833-0236 Wolk 1996 or Mayrit 165257
Caballero 2008)
(
:38:34.04,
:37.33) has been classified as M3-M4 by
Zapatero-Osorio et al. (2002) on the basis of low-resolution optical spectra
(
K). The
optical extinction is negligible (Oliveira et al. 2004), and we estimate
a luminosity of
,
based on this spectral type and the
V, R, I magnitudes (Wolk 1996).
The corresponding mass is about 0.2
,
both from D'Antona & Mazzitelli (1997) and Baraffe et al. (1998) evolutionary tracks.
Based on its location on the HR diagram,
SO587 has an age of
1 Myr, apparently slightly younger than the bulk of
the
Ori stars.
SO587 is an X-ray source, with luminosity
(Franciosini et al. 2006)
2.2 Disk properties
S0587 is detected by Spitzer at all IRAC wavelengths and at 24
with MIPS.
It shows a relatively weak excess emission, and is classified
as an evolved disk (EV, Hernandez et al. 2007). We confirm this
classification.
The IR emission is
reproduced well by a geometrically flat, optically thick disk
heated by the central star, seen at an inclination of about 40 deg.
The outer disk radius is not constrained by the existing infrared
photometry, which is limited to 24
m, as long as
AU.
The model is not unique but exploration of a large number of disk models
rules out optically thin disks,
which have different
Spitzer colors and lower luminosity than observed in SO587 (
/
)
(see Cieza et al. 2007).
Also, there is no evidence in the SED
of the large inner holes (few AUs) seen in transitional disks (Chiang & Murray-Clay 2007, and references therein), as all the
models that fit the data have inner radii
0.1 AU.
![]() |
Figure 1:
The top panel shows the spectrum in the
H |
Open with DEXTER |
2.3 Accretion rate
SO587 shows no evidence of significant accretion onto the central star.
An estimate of
can be obtained from the U band magnitude
measured by Wolk (1996).
For the spectral type and luminosity of SO587, this corresponds to an excess
U band emission of 1 mag at most.
Using the correlation between the U band excess emission and the accretion luminosity
established by Gullbring et al. (1998) for TTS, we derive
with an uncertainty of a factor 2,
i.e., about 1% of the stellar luminosity.
Since a
comparable U band excess emission may be due to chromospheric activity (White & Ghez 2001), this is
likely an upper limit to
.
The corresponding accretion rate is
/yr.
3 The optical spectrum
SO587 was observed by Sacco et al. (2008)
using
the multi-object instrument FLAMES on VLT/UT2 and Giraffe spectrograph
with the HR15N grating (6470-6790 Å, spectral resolution R=17 000).
The observations were obtained in 6 separate runs of 1 h each
in October and December 2004.
Details on the observations and data reduction, in particular on
sky subtraction, can be found in Sacco et al. (2008).
The spectra of SO587 show
H
in emission, as well as strong forbidden lines from
[NII] at 6548 and 6583 Å and [SII] at 6716 and 6731 Å
(Fig. 1).
Table 1 gives the pseudo-equivalent width (pEW) of each line
(negative values for emission lines), the FWHM (not corrected
for the instrumental resolution of about 20 km s-1), and the line luminosities.
The contribution
to the lines (both H
and the forbidden lines)
of the extended HII region due to the O9.5 star
Ori is negligible.
The line luminosities were computed using the observed R magnitude to calibrate the
continuum flux and the wavelength dependence within the band appropriate for a 3300 K star.
The luminosity is of the order of 10-5
for all the forbidden lines.
Zapatero-Osorio et al. (2002) also detected
H
and forbidden emission lines in two of their low-resolution spectra with highest quality,
but not in the other, lower-quality spectra;
when detected, the pEW of the lines are similar to our values.
We checked for evidence of line variability in the Sacco et al. spectra,
and found none.
The line profiles and intensities are very similar in each run,
with the pEW varying
10%.
In the two spectra where emission lines were detected,
Zapatero-Osorio et al. (2002) also measured the pEW of the
two oxygen lines at 6300 and 6364 Å, which are outside
our spectral range.
The corresponding luminosity is
for
the 6300 Å line and
for the
6364 Å one, similar to the [SII] and [NII] line luminosity.
Table 1: Characteristics of the emission lines.
4 Not an accretion-driven wind
![]() |
Figure 2: Distribution of the ratios [OI]6300/([SII]6716+[SII]6731) and [NII]6583/([SII]6716+[SII]6731) among the Hartigan et al. (1995) Taurus TTS with detected lines. The magenta dashed region shows the location of SO587 considering the errors on the line equivalent widths; [OI] from Zapatero-Osorio et al. (2002). |
Open with DEXTER |
The forbidden lines detected in SO587 are seen in many TTS, and are usually interpreted in the
framework of accretion-driven winds (e.g. Ferreira et al. 2006, and reference therein).
These centrifugally-driven MHD winds originate in the inner disk and move fast.
An estimate of the average mass-loss rate can be obtained from the
luminosity of the forbidden lines, following
Hartigan et al. (1995). Taking, e.g., the
[SII]6731 line, this is
The electron density







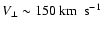


This value of
is high for a 0.2
TTS (Hartigan et al. 1995). It is
particularly high when compared to
,
as
/
10.
In TTS, this ratio is <1 and <0.1 in the majority of cases
(Hartigan et al. 1995; White & Hillenbrand 2004), in agreement with the expectations of accretion-driven jets and winds
(e.g., Shu et al. 1994; Pudritz et al. 2007).
Even taking the uncertainties on the assumptions
entering into Eq. (1) into account
(see Cabrit 2002), the
very high value of the ratio
/
makes it unlikely that the
forbidden lines
in SO587 could be emitted by an accretion-driven wind.
5 Line profiles
Additional evidence that the SO587 forbidden lines do not originate in an accretion-driven wind is provided by a comparison of the forbidden line luminosities and profiles with those of classical TTS. We first notice the strength of the [NII] lines, which are weak or absent in TTS, but comparable in luminosity to the [SII] lines in SO587. Strong [NII] lines are expected in highly ionized gas, as in HII regions or in the bow shocks at the head of jets, and are weaker than the other forbidden lines in the partially neutral wind/jets of TTS. SO587 is also unusual in having strong [SII] lines with respect to the [OI] lines (Fig. 2).
Moreover, the profiles of the SO587 [SII] and [NII] lines differ from the typical TTS line profiles.
The [SII] and [NII] are resolved in our spectra, and they show a small shift of the peak to the blue
(
),
a steep rise in the blue, and a slow decline in the
red wing.
Spatially unresolved profiles of
the [SII] 6731 Å line in a sample of accreting TTS
typically show larger peak blueshifts (
),
and significantly more emission in the blue than in the red wing
(Edwards et al. 1987; Hartigan et al. 1995). In fact,
these asymmetries are interpreted as evidence
that the disk obscures the
receding portion of the line emitting region.
The high luminosity in [NII], the low ratio [OI]/[SII] 1, and the
details of
the line profiles of SO587 are strongly reminiscent
of the properties of the Orion proplyds
(Bally et al. 1998; Henney & O'Dell 1999).
The Orion proplyds are interpreted as the
result of photoevaporation
of the disk by the hot Trapezium stars
(see, e.g. Hollenbach et al. PPIV).
The optical forbidden lines of ionized species, such as [SII] and [NII],
are formed
in the outer part of the outflow, which is ionized, heated, and shocked by the
radiation and wind of
Ori (Garcia-Arredondo et al. 2001).
6 A photoevaporating disk
We propose that
SO587 is a photoevaporating disk, which we detect in optical forbidden lines
because it is ionized
by the nearby (projected distance of 0.35 pc) O9.5 star
Ori.
Let us assume that the SO587 disk loses mass at a rate
.
Whatever the source of the photoevaporating photons (the central star or
Ori - see below),
this wind originates in the outer disk, and is slow (few
)
and neutral. However,
the high-energy (
eV) photons from
Ori ionize the outflowing gas to a distance
from SO587 roughly
Here

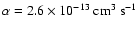







The ionization front moves away from SO587 and is closer to








In this model, the [SII] and [NII] forbidden lines originate
mostly in the ionized gas at
.
For
= 10-9
/yr, the
electron density at
from the continuity equation
(
)
is
cm-3, in good agreement
with the electron density derived from the observed ratio of the two [SII] lines (
cm-3; Sect. 4).
The [SII] 6731 Å line luminosity within the beam, computed
assuming that
all S in SII and a temperature
104 K,
is
10-5
,
in good agreement with the observations.
What is the origin of this outflow?
Both the SO587 star and Ori can, in principle, cause disk
photoevaporation, and the properties of the outflow are, qualitatively, similar.
Let us consider first the case of disk photoevaporation
due to the FUV (6 eV
13.6 eV) radiation of
Ori.
Adams et al. (2004) have computed detailed models of the photoevaporation
by an external source
of disks around stars of different masses.
At a distance of 0.35 pc, the FUV radiation field from
Ori is
(in units of the Habing radiation field; Abergel et al. 2003).
Let us assume that the SO587 disk
has mass
0.05
when the outer radius is
60 AU and
surface density
R-1.
If we take Adams et al. (2004) results for a star
of central mass 0.25
in a radiation field G0=3000(the exact value of G0 is not crucial as long as it is higher than this
value), the disk has
a first, evolutionary stage characterized by a relatively
large size and high mass-loss rates
/yr.
This phase is rapid, and the disk loses about 80% of its mass in
yr.
By that
time, the disk radius is
AU, and the mass loss rate is
10-9
/yr. In this later evolutionary stage, the
small, slowly evaporating disk has a lifetime of
yr,
comparable to the age of SO587 itself.
This scenario can explain our data, as long as SO587 stays in the
vicinity of Ori for
106 yr.
With a velocity dispersion of
km s-1 in the radial direction (Sacco et al. 2008),
the distance between
Ori and SO587 can increase to
1 pc in
106 yr,
i.e., about 3 times the present projected distance. This is still consistent with
the constraints we have set at the beginning of this section, although
marginally so.
However, it is possible that the photoevaporation of the SO587 disk is caused by
the high-energy radiation from the central star.
Recently, Gorti & Hollenbach (2008)
model the case of a low mass TTS (0.3 ),
with properties roughly comparable to those of SO587.
The trend of
with time is similar, with
an early phase when the disk is large and
/yr,
followed by a decrease in the disk radius and of
.
The phase with
/yr,
AU has a lifetime
of about
yr, which can account for the SO587 observed
properties.
In both cases,
a first period of rapid evaporation, which produces
a significant reduction of the disk size, is followed by a
phase of slower evolution, with correspondingly lower values of
.
We think that SO587 is in this late evolutionary stage, because
its disk has shrunk to 10-30 AU in radius
and is now in the last, long phase of slow photoevaporation.
SO587 shines in the optical forbidden lines of species, such as [SII] and [NII], only because it is at the right distance from
Ori at the time of our observations.
The exact values of
,
the location of the ionization front,
the dynamics of the gas, and in particular the line profiles are all
the result of the interaction of several complex physical processes,
that need to be explored.
We note, for example,
that the observed profiles of the optical forbidden lines
require a line-emitting region to be much larger than the disk, which does not seem to obscure
the receding part of the outflow.
Observationally, there are several possible tests. The disk size can be
constrained directly
by spatially resolved observations at millimeter wavelengths, but also indirectly by photometry at far-infrared wavelengths.
Also, a basic feature of externally photoevaporated disks
is the displacement between the ionization front and the star;
in SO587 this is predicted to be
0.25 arcsec,
detectable with spectroastrometric techniques or by direct
imaging from HST.
7 Discussion and conclusions
The possibility that we are detecting the final, but long-lasting stages of the dissipation of a disk by photoevaporation is intriguing.
As mentioned in Sect. 1, Ori is particularly suited to this
kind of study. First of all, the low background emission
is very favorable for detecting
objects, such as SO587, with a
mass-loss rate at least two orders of
magnitude lower than that of the Orion proplyds, and, more generally,
for studying the effects
of an external, high-energy radiation field on
jets (Andrews et al. 2004) and disks.
A second important point is the age of Ori (2-3 Myr),
significantly more than of Orion, such that disks have had enough time to evolve to the stage of low-accretion
rate, low photoevaporation mass loss.
We note that
/yrin SO587, as
expected at time
106 yr in disk evolution models where the photoevaporation
by an external source is coupled to viscous evolution
(Clarke 2007).
Some disks, such as SO587, show evidence of grain growth and settling in their SEDs, and it seems possible not only to detect old photoevaporated disks but also to relate theirproperties to those of the grains. Larger grains have two competing effects on the photoevaporation process: on the one hand, they have lower extinction in the FUV, allowing the FUV radiation to penetrate more deeply into the disk; on the other, the heating efficiency will be lower. The balance between these two competing effects needs to be explored in the model calculations, but can also be addressed observationally.
Within 0.4 pc around Ori Hernandez et al. (2007) detect about 30 objects
with all kinds of disks (Class II, Class III, and EV).
The spread of SEDs probably
reflects a varying degree of dust evolution (Dullemond & Dominik 2004;
D'Alessio et al. 2006).
We are currently carrying on a comprehensive study
of the accretion and mass-loss properties of
Ori
objects to identify
other proplyds and to address
some of the issues mentioned in this section.
Acknowledgements
We would like to thank S. Wolk and D. Hollenbach for useful discussions. We also thank the anonymous referee for comments that clarified the paper.
References
- Abergel, A., Teyssier, D., Bernard, J. P., et al. 2003, A&A, 410, 577 [NASA ADS] [CrossRef] [EDP Sciences] (In the text)
- Adams, F. C., Hollenbach, D., Laughlin, G., et al. 2004, ApJ, 611, 360 [NASA ADS] [CrossRef] (In the text)
- Andrews, S. M., Reipurth, B., Bally, J., et al. 2004, ApJ, 606, 353 [NASA ADS] [CrossRef] (In the text)
- Bally, J., Sutherland, R. S., Devine, D., et al. 1998, AJ, 116, 854 [NASA ADS] [CrossRef] (In the text)
- Baraffe, I., Chabrier, G., Allard, F., et al. 1998, A&A, 337, 403 [NASA ADS] (In the text)
- Caballero, J. A. 2008, A&A, 478, 667 [NASA ADS] [CrossRef] [EDP Sciences] (In the text)
- Cabrit, S. 2002, EAS Publ. Ser., 3, 147 [CrossRef] (In the text)
- Chiang, E., & Murray-Clay, R. 2007, NatPh, 3, 604 [NASA ADS] (In the text)
- Cieza, L. A., Padgett, D., Stapelfeldt, K. R., et al. 2007, ApJ, 667, 308 [NASA ADS] [CrossRef] (In the text)
- Clarke, C. J. 2007, MNRAS, 376, 1350 [NASA ADS] [CrossRef] (In the text)
- D'Alessio, P., Calvet, N., Hartmann, L., et al. 2006, ApJ, 638, 314 [NASA ADS] [CrossRef] (In the text)
- D'Antona, F., & Mazzitelli, I. 1997, Mem. Soc. Astr. It., 68, 807 [NASA ADS]
- Dullemond, C. P., & Dominik, C. 2004, A&A, 421, 1075 [NASA ADS] [CrossRef] [EDP Sciences] (In the text)
- Dullemond, C. P., Hollenbach, D., Kamp, I., et al. 2007, PPV, 555
- Edwards, S., Cabrit, S., Strom, S. E., Heyer, I., & Strom, K. M. 1987, ApJ, 321, 473 [NASA ADS] [CrossRef] (In the text)
- Ferreira, J., Dougados, C., & Cabrit, S. 2006, A&A, 453, 796 [NASA ADS] (In the text)
- Franciosini, E., Pallavicini, R., & Sanz-Forcada, J. 2006, A&A, 446, 501 [NASA ADS] [CrossRef] [EDP Sciences] (In the text)
- Garcia-Arredondo, F., Henney, W. J., & Arthur, S. J. 2001, ApJ, 561, 830 [NASA ADS] [CrossRef] (In the text)
- Gorti, U., & Hollenbach, D. 2008 [arXiv:0809.1494] (In the text)
- Gullbring, E., Hartmann, L., Briceño, C., et al. 1998, ApJ, 492, 323 [NASA ADS] [CrossRef] (In the text)
- Habart, E., Abergel, A., Walmsley, C. M., et al. 2005, A&A, 437, 177 [NASA ADS] [CrossRef] [EDP Sciences] (In the text)
- Hartigan, P., Edwards, S., & Ghandour, L. 1995, ApJ, 452, 736 [NASA ADS] [CrossRef] (In the text)
- Henney, W. J., & O'Dell, C. R. 1999, AJ, 118, 2350 [CrossRef] (In the text)
- Hernandez, J., Hartmann, L., Megeath, T., et al. 2007, ApJ, 662, 1067 [NASA ADS] [CrossRef] (In the text)
- Hollenbach, D. J., Yorke, H. W., & Johnstone, D. 2000, PPIV, 401 [CrossRef]
- Mayne, N. J., & Naylor, T. 2008, MNRAS, 386, 261 [NASA ADS] [CrossRef] (In the text)
- Oliveira, J. M., Jeffries, R. D., & van Loon, J. T. 2004, MNRAS, 347, 1327 [NASA ADS] [CrossRef] (In the text)
- Peimbert, M., & Rayo, J. F. 1975, RMxAA, 1, 289 (In the text)
- Pudritz, R. E., Ouyed, R., et al. 2007, PPV, 277 (In the text)
- Sacco, G., Franciosini, F., Randich, S., et al. 2008, A&A, 488, 167 [NASA ADS] [CrossRef] [EDP Sciences] (In the text)
- Shu, F. H., Nijita, J., Ostriker, E., et al. 1994, ApJ, 429, 781 [NASA ADS] [CrossRef] (In the text)
- Störzer, H., & Hollenbach, D. 1998, ApJ, 515, 669
- White, R. J., & Ghez, A. M. 2001, ApJ, 556, 265 [NASA ADS] [CrossRef] (In the text)
- White, R. J., & Hillenbrand, L. A. 2004, ApJ, 616, 998 [NASA ADS] [CrossRef] (In the text)
- Wolk, S. J. 1996, Ph.D. Thesis, State Univ. (New York: Stony Brook) (In the text)
- Zapatero-Osorio, M. R., Béjar, V. J. S., Pavlenko, Ya., et al. 2002, A&A, 384, 937 [NASA ADS] [CrossRef] [EDP Sciences] (In the text)
Footnotes
- ...
Orionis
- Based on observations collected at the European Southern Observatory, Chile. Program 074.D-0136(A).
All Tables
Table 1: Characteristics of the emission lines.
All Figures
![]() |
Figure 1:
The top panel shows the spectrum in the
H |
Open with DEXTER | |
In the text |
![]() |
Figure 2: Distribution of the ratios [OI]6300/([SII]6716+[SII]6731) and [NII]6583/([SII]6716+[SII]6731) among the Hartigan et al. (1995) Taurus TTS with detected lines. The magenta dashed region shows the location of SO587 considering the errors on the line equivalent widths; [OI] from Zapatero-Osorio et al. (2002). |
Open with DEXTER | |
In the text |
Copyright ESO 2009
Current usage metrics show cumulative count of Article Views (full-text article views including HTML views, PDF and ePub downloads, according to the available data) and Abstracts Views on Vision4Press platform.
Data correspond to usage on the plateform after 2015. The current usage metrics is available 48-96 hours after online publication and is updated daily on week days.
Initial download of the metrics may take a while.